Cephalopod camouflage: searching for good matches
Cephalopod camouflage, one of the most fascinating animal behaviors, was remarked upon already by Aristotle, around 350 BC. Many cuttlefish, octopus and squid species evolved means to imitate the substrate onto which they lie so as to escape detection by preys or predators. In a recent paper appearing in the journal Nature (June 28th, 2023), scientists at the Max Planck Institute of Brain Research and the Okinawa Institute of Science and Technology describe the great sophistication and complexity of this behavior, opening the way towards a neurobiological understanding of its mechanistic and algorithmic underpinnings.
Camouflage starts with the eyes: indeed, cephalopods use vision to estimate the essential features of a substrate’s patterning or “texture” in or on which they wish to hide. They never reproduce an exact copy of that pattern, but rather an approximation that is good enough to prevent detection (Figure 1).
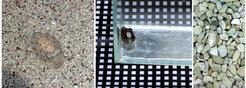
A question that follows is whether such approximations belong to a small group of “typical and good-enough patterns” that individuals might expect to encounter in their life and could produce automatically, or whether they are on the contrary varied, highly adaptive, and composed of many independently controllable features. Using a large number of printed backgrounds, high-definition movies of camouflaging cuttlefish and sophisticated quantitative analysis of hundreds of thousands of images, the scientists provide evidence in support of the latter hypothesis: although not infinitely capable, the patterns produced are far more variable, complex and high-dimensional than initially thought.
The scientists then examined the strategies used by the animals as they generate a camouflage. Cephalopods possess a unique system of specialized organs in their skin, called chromatophores, controlled by the brain. Each chromatophore contains pigment granules —the pigments come in 3 colors— in a cellular “bag” whose diameter can be controlled by a set of tiny radial muscles, themselves controlled by specialized neurons located in the brain. Each time the muscles contract, the chromatophore expands, up to about 0.3mm. Each time they relax, the chromatophore shrinks to a tiny, invisible dot. With millions of chromatophores in its skin, the cuttlefish becomes a display system, akin to a TV screen, but with colored pixels that can vary in size rather than intensity (Figure 2).
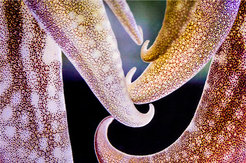
By placing the animals successively on different substrates, by measuring the size changes of hundreds of thousands of chromatophores during these camouflaging bouts, and by analyzing these datasets using modern computational methods, the scientists found that the path to the perfect camouflage resembles a wandering search with progressive error correction. In addition, when examining the population of chromatophores undergoing changes, the scientists observed that they formed groupings of tens to thousands of chromatophores, with similar size-change dynamics during a transition between camouflages. But by repeating the analysis over many similar transitions between the same pairs of backgrounds, they observed that the groupings of chromatophores was different each time. “It is a bit as if, to grab a pen in front of me, I came up with a new strategy to move my arm every time” says graduate student Theodosia Woo, joint first author of the study. These results support the idea that the animals use a form of feedback (visual or other, this is not known yet) about how they may appear, compare this to the background they want to match, and progressively try to minimize the difference between the two until they are satisfied. The whole process is intermittent, fast at the beginning, and increasingly slow as the approximation becomes better.
The scientists then examined a different display, a skin whitening called blanching, produced when the animals feel threatened. During blanching, the behavior of the chromatophores was very reproducible and direct, contrasting with the explorative aspects of camouflage pattern search. But even once in a blanched state, the animal’s chromatophores were not all equally contracted; rather they revealed very subtly the pattern that they had showed right before blanching. Correspondingly, when the blanched display disappeared, it revealed the original camouflage display again. “This suggests that camouflaging and blanching are under separate control systems that are superimposed on one another” says postdoctoral fellow Dominic Evans, responsible for this experiment.
The information gathered during these studies reveal critical information about the neural strategies used by the animals to control these very sophisticated behaviors. “One of the most interesting aspects of these animals,“ says Gilles Laurent, Director at the MPI for Brain Research and leader of the project, „is that their lineage diverged from our own and that of their fish predators over 550 M years ago, from a very primitive, worm-like common ancestor. This means that the perception of textures, which must be reasonably similar in us, fish and cephalopods —otherwise camouflage would not work— must be the result of evolutionary convergence. The brains of cephalopods are enormous by invertebrates standards, but they are built very differently from those of vertebrates. This indicates that the neural underpinnings of this form of visual perception must be similar in some algorithmic respect, despite what we know to be major differences in physical implementation. Our next objective is to discover these profound algorithmic similarities.”